Next: 3.10.3 Experiment Configuration
Up: 3.10 Global Ocean MITgcm
Previous: 3.10.1 Overview
Contents
Subsections
3.10.2 Discrete Numerical Configuration
The model is configured in hydrostatic form. The domain is discretised with
a uniform grid spacing in latitude and longitude on the sphere
, so
that there are ninety grid cells in the zonal and forty in the
meridional direction. The internal model coordinate variables
and are initialized according to
Arctic polar regions are not
included in this experiment. Meridionally the model extends from
to
.
Vertically the model is configured with twenty layers with the
following thicknesses
(here the numeric subscript indicates the model level index number, ) to
give a total depth, , of
.
The implicit free surface form of the pressure equation described in Marshall et. al
[39] is employed. A Laplacian operator, , provides viscous
dissipation. Thermal and haline diffusion is also represented by a Laplacian operator.
Wind-stress forcing is added to the momentum equations in (3.36)
for both the zonal flow, and the meridional flow , according to equations
(3.30) and (3.31).
Thermodynamic forcing inputs are added to the equations
in (3.36) for
potential temperature, , and salinity, , according to equations
(3.32) and (3.33).
This produces a set of equations solved in this configuration as follows:
 |
 |
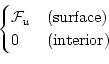 |
(3.36) |
 |
 |
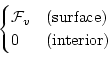 |
(3.37) |
 |
 |
0 |
(3.38) |
 |
 |
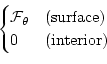 |
(3.39) |
 |
 |
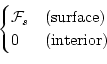 |
(3.40) |
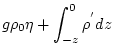 |
 |
 |
(3.41) |
where
and
are the zonal and meridional components of the
flow vector, , on the sphere. As described in
MITgcm Numerical Solution Procedure 2, the time
evolution of potential temperature, , equation is solved prognostically.
The total pressure, , is diagnosed by summing pressure due to surface
elevation and the hydrostatic pressure.
3.10.2.1 Numerical Stability Criteria
The Laplacian dissipation coefficient, , is set to
.
This value is chosen to yield a Munk layer width [1],
|
|
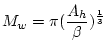 |
(3.42) |
of
km. This is greater than the model
resolution in low-latitudes,
, ensuring that the frictional
boundary layer is adequately resolved.
The model is stepped forward with a
time step
for thermodynamic variables and
for momentum terms. With this time step, the stability
parameter to the horizontal Laplacian friction [1]
|
|
 |
(3.43) |
evaluates to 0.16 at a latitude of
, which is below the
0.3 upper limit for stability. The zonal grid spacing is smallest at
where
.
The vertical dissipation coefficient, , is set to
. The associated stability limit
 |
|
|
(3.44) |
evaluates to for the smallest model
level spacing (
) which is again well below
the upper stability limit.
The values of the horizontal ( ) and vertical ( ) diffusion coefficients
for both temperature and salinity are set to
and
respectively. The stability limit
related to will be at
where
.
Here the stability parameter
 |
|
|
(3.45) |
evaluates to , well below the stability limit of
. The
stability parameter related to
 |
|
|
(3.46) |
evaluates to for
, well below the stability limit
of
.
The numerical stability for inertial oscillations
[1]
 |
|
|
(3.47) |
evaluates to for
, which is close to
the upper limit for stability.
The advective CFL [1] for a extreme maximum
horizontal flow
speed of
 |
|
|
(3.48) |
evaluates to
. This is well below the stability
limit of 0.5.
The stability parameter for internal gravity waves propagating
with a maximum speed of
[1]
 |
|
|
(3.49) |
evaluates to
. This is close to the linear
stability limit of 0.5.
Next: 3.10.3 Experiment Configuration
Up: 3.10 Global Ocean MITgcm
Previous: 3.10.1 Overview
Contents
mitgcm-support@dev.mitgcm.org
Copyright © 2002
Massachusetts Institute of Technology |
 |
|